
November 2022
Food irradiation is the physical treatment of food with high-energy ionising radiation for the purpose of prolonging shelf life and prevent food-borne diseases in meat, poultry and seafood. Irradiation achieves this by destroying micro-organisms, viruses, bacteria or insects; preventing germination and sprouting of potatoes, onions and garlic and slowing down the ripening and ageing of fruit and vegetables.
Irradiation can reduce the risk of food poisoning, control food spoilage and extend the shelf-life of foods without detriment to health and with minimal effect on nutritional or sensory quality. This view has been endorsed by international bodies such as the World Health Organisation (WHO), the Food and Agricultural Organisation (FAO) and Codex Alimentarius.
Irradiated food should not be confused with the possible radioactive contamination of foods following nuclear incidents. Irradiated food exposed to ionising radiation sources does not become radioactive itself. The amount of energy that is used in effective food irradiation is low compared to cooking. Multiple safety assessments by governmental authorities have confirmed that irradiated food is safe to consume.
Food irradiation, when carried out under conditions of Good Manufacturing Practice (GMP), is an effective and efficient food processing method, is judged to be safe based on extensive available scientific evidence. Irradiation should not be used as a substitute for good hygienic and good manufacturing practices or good agricultural practices. For the irradiation of any food, the minimum absorbed dose should be sufficient to achieve the intended technological purpose and the maximum absorbed dose should be less than that which would compromise consumer safety, wholesomeness or would adversely affect structural integrity, functional properties, or sensory attributes.
Many processing methods have been developed to help prevent food spoilage and improve safety. The traditional methods of preservation, such as drying, smoking and salting have been supplemented over time by pasteurisation (by heat), canning (commercial sterilisation by heat), refrigeration, freezing, the addition of chemical preservatives and use of irradiation.
Irradiation of food is not new. Interest in treatment of food with ionising radiation was shown in Germany in 1896 (Stewart, 2004(a)) and food irradiation has been applied since the early 1920s. In the 1950/60s the US Army Natick Soldier Center (NATICK) experimented with both low dose and high dose irradiation for military rations, whilst at the same time, the United Kingdom (UK) authorities Low Temperature Research Station programme concentrated on low dose pasteurisation (Hannan, 1955). Irradiation is extensively used in the medical field for sterilising instruments, dressings, etc. and for sterilisation of packaging used for a range of consumer products including medicines, cosmetics and food.
Codex Alimentarius General Standard for Irradiated Foods CXS 106-1983, Rev.1-2003 Available online: https://www.fao.org/fao-who-codexalimentarius/codex-texts/list-standards...
European Food Safety Authority; Statement summarising the Conclusions and Recommendations from the Opinions on the safety of Irradiation of Food adopted by the BIOHAZ and CEF Panels. EFSA Journal 2011; 9(4): 2107. [155 pp.] doi:10.2903/j.efsa.2011. 2107. Available online: www.efsa.europa.eu/efsajournal
History and future of food irradiation, József Farkas, Csilla Mohácsi-Farkas, Trends in Food Science & Technology, Volume 22, Issues 2–3, 2011, Pages 121-126, ISSN 0924-2244, https://doi.org/10.1016/j.tifs.2010.04.002.
Subject Interest Area:
- Food Safety
- Food Hygiene
- Food Processing
Keywords
- Food Safety
- Microbiological Safety
- Food Irradiation
- Food Spoilage
- Shelf Life
Irradiation refers to the process by which an object is deliberately exposed to a certain type of radiant energy known as ionising radiation[1]. Radiant energy comes from a variety of sources producing radiation with different wavelengths and degrees of power. The degree of radiation penetration into materials is dependent on its wavelength. Light (including ultraviolet, visible and infrared light), radio waves, and microwaves are all types of non-ionising radiation. Ionising radiation on the other hand carries more energy that non-ionising radiation and can produce charged particles (ions) in matter, hence the name. When ionising radiation penetrates a material; this type of radiant energy is absorbed in part or completely by the treated material. The absorbed radiant energy causes excitation, ionisation and chemical alteration of the treated material components. Once the irradiation treatment stops, the material quickly loses this absorbed energy in the same way that cooked food quickly cools down.
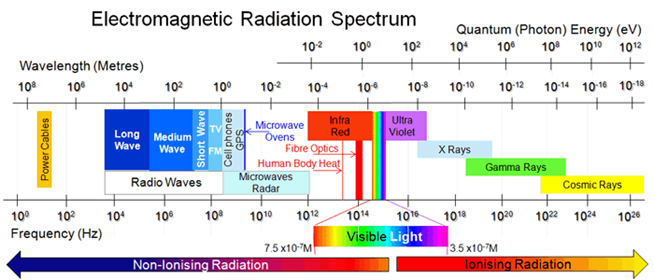
Free radicals formed upon irradiation with ionising radiation are highly reactive and very short-lived, so short-lived that they cannot be detected in water-containing food almost immediately after it has been irradiated. In all but very dry matter, such as bone or shell, small amounts of substances are formed upon treatment with ionising radiation, some of which are highly active but transient free radicals, others of which are useful as markers which can be used to determine whether or not the food has been irradiated. It is the action of the transient free radicals, which accounts for much of the effects (killing pathogenic bacteria, extending shelf-life etc.) of irradiating food.
Ionising radiation is therefore effective for sterilisation and reducing viable presence of pathogenic microorganisms, whether used in irradiation of medical instruments, packaging or foods, because high-speed electrons, gamma rays and X-rays, and the free radicals they produce, denature DNA (deoxyribonucleic acid) and RNA (ribonucleic acid). Disruption of the weak hydrogen bonds between nucleotides prevents DNA and RNA replication and causes cell death while exerting minimal effects on non-living tissue. In much the same way, ionising radiation can slow down cell-based processes such as ripening of fruit, which would lead to premature decay or sprouting of vegetables.
The unit of ionising radiation absorbed dose is a Gray (Gy). One Gray corresponds to the absorption of one joule of energy in a mass of one kilogram (1Gy = 1J/kg). The Gray has superseded the older unit, the rad (1Gy = 100 rad). The length of time the material is exposed to the ionising energy coupled with the strength of the source determine the irradiation dose, measured in Gray (Gy), or kiloGray (kGy).
Food irradiation is the exposing food to a carefully controlled dose of ionising radiation in order to, among other things, control foodborne pathogens, reduce microbial load and insect infestation, inhibit the germination of root crops, and extend the durable life of perishable produce. Irradiated food is not radioactive and should be safe to consume, providing the irradiation treatment is performed such that the food never comes into contact with the ionising radiation source, performed under controlled conditions using an approved source and at a licensed approved irradiation premises.
A fundamental principle in the use of radiation processing is that irradiation should never be used as a substitute for good manufacturing practices (GMP) in the control of food safety and quality. It is unacceptable to attempt to irradiate an unfit or contaminated foodstuff or out of date food to render it fit to consume. Food irradiation can however play a significant role where GMP and prerequisite controls are not sufficiently capable of delivering the level of microbiological quality required. It can offer a tool for food decontamination from microbial loading and for controlling pest infestation of foods and of plant materials more broadly. The increased globalisation of trade has increased the likelihood of invasive species being introduced to new countries and climate change has increased the likelihood that invasive species will survive in these new environments. Irradiation can prevent the reproduction of adult insects and their larvae and can as such be an effective tool to address plant health risks. Irradiation is however not suitable for on the spot treatments when infestations are discovered, due to the specific infrastructure and packaging required to carry out irradiation.
Currently, radiation sterilisation of agricultural and food products is carried out in more than 60 countries. According to the UN FAO, more than 200,000 tons of irradiated products are annually produced only in Europe. For many types of products, the optimal modes of radiation sterilisation were determined, long-term studies of their suitability and harmlessness for use were carried out, and adequate radiation equipment was created. Decontamination of spices, herbs and condiments remains the single largest application of irradiation. In recent years the market for irradiated fresh and processed meat has become firmly established in several countries including China and the USA. At least 10 countries have recently established bilateral agreements for trade in irradiated fresh fruits and vegetables using phytosanitary irradiation. Irradiated fresh produce volumes now exceed 20,000t per year (Ferreria et al 2017).
More than 100 years of research have gone into the understanding of the safe and effective use of irradiation as a food safety method, more than any other technology used in the food industry today, even canning (Scott Smith and Pillai, 2004). The safety and efficacy of the technology has been repeatedly considered and judged acceptable on available evidence. This has resulted in international bodies including the World Health Organisation (WHO), the United Nations Food and Agriculture Organisation (FAO), the International Atomic Energy Agency (IAEA) and Codex Alimentarius commending food irradiation as a process supporting food hygiene and food safety.
A maximum overall average dose of 10 kGy was considered adequate for the majority of food applications. Over 50 countries have given approval for the irradiation of over 60 foods and food products on either a conditional or unconditional basis. Research carried out worldwide has shown that irradiation of food is a safe and effective way to kill bacteria in foods and extend its shelf life. Food irradiation has been examined thoroughly by joint committees of the WHO, FAO, by the European Community Scientific Committee for Food, the United States Food and Drug Administration (FDA) and by a House of Lords committee in the UK. In 2011, the European Food Safety Authority (EFSA) reviewed the evidence and reasserted the opinion that food irradiation is safe (EFSA, 2011). They concluded:
-
- that there are no microbiological risks for the consumer linked to the use of food irradiation and its consequences on the food microflora
- that most of the chemical substances formed during irradiation were also formed in food that has been subjected to other processing treatments and that the quantities in which they occur in irradiated food were not significantly higher than those being formed in heat treatments.
The recent European Union (EU) evaluation of the EU legal framework on food irradiation (EC, 2021), restates that food irradiation is an established food decontamination technique and when integrated into an overall food safety management program that includes Good Agricultural, Manufacturing and Hygienic Practices and HACCP, and depending on the dose applied, food irradiation can contribute to improved consumer safety by reducing food-borne pathogens. Concerns raised by consumer associations and the European Parliament that food irradiation may be misused by food business operators to mask poor hygiene in production processes, were addressed. The EU confirmed that such practice would be in contradiction with the food hygiene regulations.
Concerns regarding the toxicity of the 2-alkylcyclobutanone (2-ACBs) group of radiolytic compounds have been identified. New studies carried out confirm that the ingestion of 2-ACBs through irradiated foods is unlikely to affect the human health but suggest that the determination of chronic toxicity by long-term exposure to low concentrations of 2-ACBs has to be evaluated more clearly to determine if these compounds are safe to human. Considering that only a very limited quantity of food is irradiated in Europe currently, the current view is that there is not an immediate cause for concern.
A concern was also raised by one national competent authority, in the 2021 EU evaluation, about possible migration of elements from packaging and into food in the context of food irradiation. It is described in the scientific literature that for pre-packaged foods undergoing irradiation, packaging made from polymers are susceptible to radiation effects. The extent of these effects differs depending on the polymer. This is not a new issue, and packaging materials used for foods undergoing irradiation must be suitable for the purpose. As new packaging materials are developed, they should be tested before use in the irradiation process.
Foods sterilised by high dose irradiation (>25 kGy), cold sterilisation as opposed to thermal sterilisation (canning), have been consumed by astronauts in the NASA space shuttle programme because of their superior quality and variety, compared to foods treated by other preservation technologies. There is a small but increasing demand for sterile products for immunocompromised patients as well as for niche markets, such as the military, campers or disaster victims where a long shelf life at ambient temperatures is required. High dose sterile foods may be prepared under medical supervision for immunocompromised patients without labelling. A research programme on Irradiated Foods for Immuno-compromised Patients was coordinated by the IAEA (IAEA, 2010).
Irradiation is very effective against living organisms which contain DNA and/or RNA but does not cause any significant loss of macronutrients. Proteins, fats and carbohydrates undergo little change in nutritional value during irradiation even with doses over 10 kGy, though there may be sensory changes. Similarly, the essential amino acids, essential fatty acids, minerals and trace elements are also unaffected. There can be a decrease in certain vitamins (particularly thiamin), but these are of the same order of magnitude as occurs in other manufacturing processes such as drying or canning (thermal sterilisation) (WHO, 1994; FAO/IAEA/WHO, 1999; EFSA, 2011). In some cases, vitamin levels can be found to increase. Niacin content of bread from irradiated flour was found to be 17% more than the non-irradiated control flour (Diehl, 1991) and an increase in the niacin content (24%) and riboflavin content (15%) in pork chops after irradiation was reported in another study (Fox et al, 1989).
The irradiation process is not suitable for all products. Foods with high fat contents, such as fatty fish and some dairy products, can develop off-odours and tastes due to the acceleration of rancidity, even at relatively low doses. Loss of firmness can occur with some fruits and vegetables. Foods with a high protein content, such as meat and poultry, can suffer from changes in flavour and odour after irradiation at ambient temperatures but these changes can be reduced by irradiating at chill temperatures and minimised by irradiating at frozen temperatures. For fresh ground beef with a high fat content and for fatty pork products, the maximum dose should not exceed 2.5 kGy to prevent rancidity. Liquid and dry eggs can tolerate doses in excess of 3 kGy, but for shell eggs a 2 kGy dose can cause deterioration of the yolk sac membrane. Milk develops an off-flavour at relatively low doses, but various cheeses show good tolerance at doses up to 3 kGy (Diehl, J.F., 1983). All these changes are minimised by irradiating at chill or frozen temperatures, and, in addition, many changes can be minimised by careful choice of suitable packaging systems coupled with controlled gas atmospheres.
With respect to dose, populations of pathogenic and spoilage microorganisms in food products vary significantly in their radiosensitivity. For example, the lethal dose for mould fungi Penicillium expansum is 100–200 kGy, and for Pullularia pullulans, it is 2000 kGy. For solving this problem, the IAEA special commission proposed several different modes for radiation treatment for sterilisation: radicidation (4 - 6 kGy), radurisation (6 - 10 kGy), and radappertisation (10 - 50 kGy) (Obodovskiy, 2019).
Radurisation, from the Latin ‘radiare’ (radiate) and ‘durare’ (prolong), is a radiation treatment of food products for increasing the duration of storage, in doses, leading to limited suppression of certain pathogenic for humans’ microorganisms. For example, treatment of potatoes and onions with 0.05 - 0.15 kGy to inhibit germination; treatment of grains, fruits with 0.15 - 0.50 kGy for pest control; treatment of fruits and vegetables with 0.5 - 1.0 kGy to slow maturation, treatment of strawberries and vegetables with 5 - 3.0 kGy to extend the shelf life, and treatment of fresh and frozen chicken meat, shrimps, frog's feet with 2.0 - 5.0 kGy for the destruction of pathogenic parasites and microorganisms.
Radicidation from the Latin ‘radiare’ (radiate) and ‘ocsidere’ (kill), is a radiation treatment for the purpose of selective suppression of microorganisms of any type, e.g. Salmonella, Trichinella. For example, treatment of food products with 2 - 10 kGy to improve the technological properties of a number of foods.
Radappertisation, by the name of Nicolas Appert, the inventor of thermal sterilisation method of food products, is carried out for industrial sterilisation of food products for complete destruction of microorganisms, as it occurs in the thermal sterilisation in canned food manufacturing. For example, treatment of food additives and some ingredients, e.g. spices with 10 – 50 kGy for disinfection and treatment of meat, meat products, dietetic products for sick people with 30 - 50 kGy for sterilisation.
The absorbed doses permitted for food irradiation are usually defined in regulatory requirements where the ionising radiation sources, irradiation conditions and doses for food irradiation are prescribed for specific types of foods and purposes. Doses of irradiation that have been approved vary from 0.05 kGy, to inhibit sprouting in white potatoes, to 30 kGy, to sterilise herbs and spices.
Three principal types of radiation source can be used in food irradiation according to the Codex Alimentarius General Standard 106 (Codex, 2003):
(a) gamma radiation from radionuclides such as 60Co or 137Cs
(b) machine sources of electron beams with energies up to 10 MeV
(c) machine sources of bremsstrahlung (X-rays) with electron energies up to 5 MeV.
The choice of irradiation method and the dose applied will depend on the material needing to be treated and the intended purpose for treatment. The effectiveness of processing of food by ionising radiation depends on proper delivery of absorbed dose and its reliable measurement. It is important that the dosimetry techniques used for dose determination are carried out accurately and that the process is monitored.
Food irradiation is carried out in specific containment areas applying the necessary shielding to prevent the escape of ionising radiation and to avoid exposure of personnel. In automated irradiation facilities, conveyors or other carrier systems move the products to be irradiated at a predetermined speed or rate to deliver the necessary dosage. At no time during the irradiation process does the food come into contact with the radiation source. By using gamma rays, X-rays up to 5 MeV or electron beams up to 10 MeV, none of these kinds of ionising radiation have energy levels sufficient to induce radioactivity in the irradiated food.
Gamma rays and X-rays can penetrate food to a depth of about 1 metre; whereas an electron beam can only penetrate foods to a depth of about 3cm. Thus, to treat the surface or a thin layer of a food, one would usually choose to use an electron beam. These are easy to produce electronically but they do not have deep penetrating power. For situations where only shallow penetration is needed and where rapid conveyor speeds can be used, high power electron beams may provide a higher output at lower cost per unit of product when large amounts of product are involved. To treat a bulky product such as an entire sack of spices, one would choose gamma rays or X-rays. Irradiation using a gamma ray source is possible from two sides either by turning the process load or by irradiation from two sides of a plaque source, often used to increase the dose uniformity in the process load (Olivieira, et al., 2000; Saylor & Jordan, 2000).
Food once irradiated, can be prone to re-contamination unless appropriately packed. Pre-packaging is an integral part of the process when irradiation is intended to control microbiological spoilage or insect infestation. The packaging provides the technical function of protecting the food product from external conditions such as prevention of moisture uptake or loss, maintenance of an atmosphere other than air, protection from mechanical damage and from contamination from physical, chemical and microbial hazards. Since packaging materials are therefore also exposed to irradiation during the treatment, these materials must also satisfy additional requirements such as resistance to irradiation with respect to its functional properties and as a suitable food contact material. It therefore should not transmit toxic substances into food nor impart any off odour to the products. It is possible that irradiation might either affect barrier properties or that radiolytic products formed in the packaging might be absorbed into the product. Consideration should also be given to the suitability of components such as absorbent pads, adhesives and printing inks, especially in minimising the risk of taint from low levels of radiolytic products. Increasingly, packaging materials for use in aseptic processing lines in the food, pharmaceutical and cosmetic industry are now being sterilised by ionising radiation. The evaluation of the suitability of new packaging materials for irradiation either when used to pack foods for irradiation or for direct irradiation for sterilisation of packaging priori to use is described in Komolprasert (2007).
A number of applications for food irradiation have been identified, aimed at improving microbiological safety and reducing food spoilage. Examples of application areas include:
Insect contamination/infestation - low dose (less than 1 kGy) irradiation for insect control (for instance in grain and grain products) where a dose of 150-700Gy is sufficient.
Poultry and poultry products, including mechanically recovered meat - to reduce numbers of Salmonella, Campylobacter and other food poisoning bacteria. Doses of up to 3 kGy (fresh) and up to 7 kGy (frozen) have been recommended. In 2012, the Food and Drink Administration (FDA) extended the maximum dosage for poultry in the USA to 4.5 kGy.
Red meats, including particularly comminuted meat such as used in hamburgers - to reduce numbers of E.coli O157:H7 and other food poisoning bacteria. Doses of up to 4.5 kGy (fresh) and up to 7 kGy (frozen) have been recommended. The irradiation of meat in the USA was extended by the FDA in 2012 to cover unrefrigerated meat.
Frogs’ legs, especially in Belgium, France, The Netherlands and Finland - to reduce numbers of food poisoning bacteria
Dried herbs and spices - to reduce overall levels of contaminating pathogenic microorganisms generally and to reduce or eliminate food poisoning bacteria in particular. Doses up to 10 kGy have been recommended. Herbs and spices are the food materials most commonly irradiated. These raw agricultural products, grown and harvested by traditional methods are only processed by mild drying which does not reduce the level of microbes present. Alternative methods to reduce microbial numbers have used chemicals, such as ethylene oxide and methyl bromide that are now considered dangerous to humans and/or the environment. This has led to a large trade in steam flash pasteurised spices, but which can result in flavour losses.
Some types of seafood, in particular warm water shrimp/prawns and other shellfish, to improve their microbiological safety - doses up to 3 kGy have been recommended. Low doses (<3 kGy) eliminate 90 - 95% of spoilage organisms, resulting in an improvement in shelf-life and eliminate all vegetative bacterial pathogens. Shrimp in ice have a shelf life of 7 days and treating with 1.5 kGy adds another 10 days. 1 kGy eliminates both E.coli and Vibrio spp. in oysters without detracting from their raw quality. Oyster meats treated with 2 kGy have a shelf-life of 21 to 28 days under refrigeration, compared to 15 days for their non-irradiated counterpart (Komolprasert, V 2002). The Vibrios spp. most common in crustaceans and bivalve molluscs (V. vulnificus and V. parahaemolyticus), are very sensitive to irradiation and they are reduced to below detectable levels with a treatment of only 300 Gy.
Certain fruits and vegetables in order to reduce the numbers of microorganisms, particularly those that cause spoilage - doses of up to 2 kGy have been recommended. Irradiation has been shown to have minimal effect on flavour, aroma and colour but can have an adverse effect on texture (Komolprasert,V, 2002). Irradiation in combination with modified atmosphere packaging (MAP) exerts a useful synergistic effect (Grant, I.R. and Patterson, M.F., 1991) although this combination processing is not allowed in some countries. Irradiation of onions, garlic, mung beans and tamarind is commercially viable in Thailand. Irradiation is also useful in combating rice weevil (Sirohilus oryzae) and lesser grain borer (Rhyzopertha dominice). It is particularly effective against internal feeders. Only a few species are internal feeders, but larvae and pre-emergent pupae present the greatest challenge.
Leafy vegetables, especially salad leaves - the USA approved the use of irradiation of spinach and iceberg lettuce in 2008 on grounds of safety and shelf life extension. This followed several cases of food poisoning attributed to E.coli contamination, including fatalities.
Bulbs and tubers, such as potatoes and onions - for various purposes. Doses of less than 1kGy have been recommended. Potatoes have been irradiated in Japan for over 26 years to prevent sprouting (IAEA 1991), and sweet potatoes are irradiated in Hawaii to control insect infestation (Follett and Weinert, 2012).
Sterilise foods with longer shelf life or for specific purposes, such as ready meals, for special medical diets, emergency or space diets - these foods are irradiated by doses of 45 kGy to render the foods microbiologically sterile. The irradiation is carried out under frozen conditions to minimise adverse sensory effects. The foods can be subsequently distributed unrefrigerated. Shelf-stable meat dishes have been prepared in South Africa since 1989 for both military and non-military uses (WHO, 1999), but has since been discontinued on cost grounds (Amanda Minnaar, personal communication).
In 2003, the Codex Alimentarius Commission (a body established by the United Nations Food and Agriculture Organisation (FAO) and the World Health Organisation (WHO) in 1963 to develop harmonised international food standards), published two key documents in the field of food irradiation: the Codex General Standard for Irradiated Foods and the Recommended International Code of Practice for Radiation Processing of Food. Other important documents, developed by the IAEA (International Atomic Energy Agency) together with the FAO and the IPPC (International Plant Protection Convention), are the Guidelines for the Use of Irradiation as a Phytosanitary Measure and the Phytosanitary Treatments for Regulated Pests, the second of which includes fifteen irradiation treatments for thirteen specific insect pests, one for all fruit flies and one for three types of mealybugs. Codex Alimentarius has developed standards and a code of practice to effectively apply the irradiation technology to improve food safety, together with guidance on the labelling of irradiated foods.
The current upper limit of 10 kGy is insufficient to achieve sterility. This led a Joint FAO/IAEA/WHO Study Group on High-Dose Irradiation to request the International Consultative Group on Food Irradiation, to petition the Codex Secretariat to remove the upper dose limit of 10 kGy by revising the General Standard. This recommendation was based on the usefulness of effectively eliminating the more resistant spores of proteolytic strains of Clostridium botulinum as well as all vegetative organisms while neither compromising nutritional values nor resulting in any toxicological hazard. This process of cold sterilisation is analogous to canning (thermal sterilisation) the products of which have been safely consumed for well over a century. The conclusion reached by the Joint Study Group was that food irradiated to any dose appropriate to achieve the intended technological objectives is both safe to consume and nutritionally adequate. They also advised that no upper dose limit need be imposed. The Joint Study Group concluded that appropriate steps need to be taken to establish the technological guidelines implied by these conclusions and then to communicate them through Codex Alimentarius standards in order to achieve global standardisation. The revised Codex General Standard (2003) for Irradiated Foods now reads, “the maximum absorbed dose delivered to a food shall not exceed 10 kGy, except when necessary to achieve a legitimate technological process”. However, the EU SCF disagreed with this view and saw inadequate reason to raise the upper limit of 10 kGy. The FDA in the US allows frozen meat for NASA to be irradiated to sterility with a minimum dose of 44 kGy (http://www.cfsan.fda.gov/~dms/opa-fdir.html [21]).
Separately, an ISO standard has been developed on food irradiation to support the development, validation and routine control of the process of irradiation using ionising radiation for the treatment of food. ISO 14470:2011 specifies requirements for the development, validation and routine control of the process of irradiation using ionising radiation for the treatment of food and establishes guidelines for meeting the requirements. ISO 14470:2011 covers irradiation processes using the radionuclides Co60 or Cs137, electron beams or X-ray generators. The requirements given in ISO 14470:2011 are the minimum necessary to control the food irradiation process. It does not specify requirements for the primary production and/or harvesting, post-harvest treatment, storage and shipment, and packaging for foods that are to be irradiated. Only those aspects of the food production directly related to the irradiation process that may affect the safety or quality of the irradiated food are addressed. ISO 14470:2011 does not specify requirements for occupational safety associated with the design and operation of irradiation facilities, or the measuring or inspection devices that utilise ionising radiation.
There are international standards supporting best practice application of food irradiation. Governments determine their own national approaches to the use of food irradiation with food irradiation permitted as a treatment with defined conditions of authorised use in many countries (Roberts, 2016). More than 50 countries permit food irradiation under prescribed conditions for over 60 types of food products. However, these permissions vary between countries. Therefore, careful consideration should be also given to the application of food irradiation from a regulatory compliance point of view. Food irradiation applications are not necessarily approved by regulators in a specific market of sale. Irradiation of any foodstuff will need to be reviewed to confirm that the proposed irradiation source, dose, site performing the irradiation and types of food are permitted in law.
The USA, China, The Netherlands, Belgium, Brazil, Thailand and Australia are among the leaders in adopting the technology. Food irradiation, as defined in legislation in the UK[1] and EU[2], currently only addresses ionising radiation treatments from sources of gamma rays, electrons or X-rays[3]. In the EU and UK, food irradiation is permitted for certain food categories only, e.g. fruit, vegetables, fish, poultry, providing it is necessary, presents no hazard, is beneficial to consumers and is not used as a substitute for good manufacturing practice. The lists of foods permitted to be irradiated differ in each Member State, and from EU to UK, so should be carefully checked to ensure compliance. See document 52009XC1124(02) https://eur-lex.europa.eu/oj/direct-access.html , List of Member States’ authorisations of food and food ingredients which may be treated with ionising radiation (according to Article 4(6) of Directive 1999/2/EC of the European Parliament and of the Council on the approximation of the laws of the Member States concerning foods and food ingredients treated with ionising radiation), which cancels and replaces the text published in Official Journal C 112 of 12 May 2006, p.6.
Labelling is required in all EU member states, and in the UK, such that irradiated foods must be labelled as either ‘treated with ionising radiation’ or ‘irradiated’. This is intended to ensure that consumers are fully informed about whether foods, or ingredients within them, have been irradiated.
Currently regulations on food irradiation in the EU are not fully harmonised. Directive 1999/2/EC establishes a framework for controlling irradiated foods, their labelling and importation, while Directive 1999/3 establishes a positive list of foods which may be irradiated and traded freely between Member States. In addition, individual EU member states have additional national authorisations of food and food ingredients which may be treated with ionising radiation[4].
There are a range of analytical test methods which can detect whether a food has been irradiated. These analytical tests may be used to enforce food irradiation authorisation and labelling requirements. The consumers’ ability to make informed choices mainly depends on accurate labelling. Analytical methods that can discriminate between irradiated and non-irradiated foods are required if labelling regulations are to be enforceable.
The changes known to occur in irradiated foods are minimal and often similar to those occurring in foods subjected to other processes, which has made the development of sufficiently sensitive tests difficult. In all but very dry matter, such as bone or shell, small amounts of substances are formed, some of which are highly active but transient free radicals, others of which are useful as markers, which can be used to determine whether or not the food has been irradiated. It is the action of the transient free radicals which accounts for much of the effects (killing pathogenic bacteria, extending shelf-life etc.) of irradiating food.
Analytical test methods used for the detection of irradiated food based on physical, chemical, biological, and microbiological changes in food products during irradiation have been successfully developed (Chauhan et al 2009). These are based on the detection of physical, chemical and microbiological changes that can occur in irradiated food.
Physical methods measure the effect of the radiation generated radicals or trapped electrons in solid materials. They include:
Electron spin resonance spectroscopy (ESR) based on quantum theory and detects the irradiation produced long-lived paramagnetic active sites of the free radicals in the organic and inorganic complexes possessing a transition metal ion.
Thermoluminescence (TL) where the emission of light from irradiated solids can be determined based on the effect that a small portion of absorbed radiation energy stored at low temperature is emitted in the form of light when heated.
Photoluminescence (PL). This technique is analogous to thermoluminescence, except using light rather than heat to release the trapped energy. This method may in principle be applied to detect irradiation of any foods, which contain mineral debris, especially silicate mineral and bioinorganic material such as calcite, which originate from shells or exoskeletons, or hydroxyapatite from bones or teeth.
Chemoluminescence (CL). Also, very similar to thermoluminescence and refers to emission of light on dissolution of a solid substance in liquid media where emission of electromagnetic radiation as light takes place when trapped energy is liberated by the addition of chemicals. Thus, it is not strictly a physical effect.
Viscosity measurement. The viscosity of foodstuffs is largely dependent on the content and structure of polysaccharides, proteins, gums, and so on, which may undergo alteration by irradiation. The irradiation-induced change in these molecules is mainly chain-breaking or hydrolysis, resulting in changes in viscosity due to increased cross-linking or decreased chain length.
Electrical impedance measurement. The membrane of living tissues has selective permeability to transport ions and . membrane properties can be changed by changing ion concentration. This tool can be used for the detection of irradiation for food samples having intact tissue such as fresh produce.
Chemical methods measure the effect of the radiation on biochemical processes within the irradiated foodstuff. They include:
Changes in lipids Triglycerides in fat break down upon irradiation in the alpha and beta positions to produce radiolytic products such as 2-alkyl cyclobutane which is widely used as a radiolytic marker. The formation of major radiolytic products increases linearly with dose and temperature of irradiation and can be used to indicate the severity of irradiation treatment.
Ortho-tyrosine. Most food proteins contain aromatic amino acids which react with hydroxyl radicals formed during the radiolysis of water and form ortho- and meta-tyrosine. These isomers of tyrosine are not naturally present
Estimation of gas evolution. Estimation of gases, such as carbon monoxide, hydrogen sulphide, hydrogen, ammonia, and others from food can also be utilised for the detection of irradiation processing
DNA methods detect the changes in this major cellular target for ionising radiation. Irradiation resulting in DNA damage is responsible for the inactivation of living microorganisms, inhibition of microbe growth, and other lethal effects. There are 3 types of major changes that take place in DNA upon exposure to ionising radiation, namely double-strand breaks, single-strand breaks, and base damage. Methods to detect these DNA changes include:
Agarose electrophoresis of mitochondrial DNA targets radiation-specific breakage of DNA isolated from cells with enzymatically ruptured DNA. Mitochondrial DNA (mDNA) is protected from enzymatic reactions due to the presence of mitochondrial walls, but it is not protected from ionising radiation damage. Therefore, it is assumed that any detected mDNA breakage is specific to radiation.
Immunological detection of modified DNA bases. On irradiation, a proportion of thymine (nucleotide found in NDA) is converted to dihydrothymidine (DiHT) which can be used as a potential internal marker for radiation-processing.
Biological methods leverage the fact that ionising radiation causes destruction or changes in microbial load in foodstuffs. These methods will often be comparative or used as screening methods and look at for example at the reduction of overall microbial load or bacterial population, the viable cell count when compared to the total number of organisms present ( e.g. direct epifluorescent filter technique combined with aerobic plate count (DEFT/APC)), the rate of growth such as sprout inhibition, root growth and shoot elongation and the viability of seed germination.
The most useful and widely used methods include electron spin resonance spectroscopy (ESR), luminescence methods (thermo-luminescence – TL), photo stimulated luminescence (PSL) and the detection of long-chain volatile hydrocarbons and 2-alkylcyclobutanones. The luminescence methods are widely applicable and make use of mineral grains that are present in many foods including herbs, seasonings, spices, fruits, vegetables and shrimp. ESR can be used to detect free radicals captured in the dry parts of foods such as bones, seeds and shells, while methods based on detection of hydrocarbons and 2-alkylcyclobutanones are applicable to foods containing fat, such as meat. These methodologies complement each other and allow discrimination between irradiated and non-irradiated products in a wide variety of foods, although uncertainties in the interpretation of results from different methods can occur.
A range of analytical methods for the detection of irradiated foods have been standardised by the European Committee for Standardisation (CEN). These have also been adopted by the Codex Alimentarius Commission as General Methods in CXS 231-2001 and are referred to in the Codex General Standard for Irradiated Foods[1] CXS 106-1983 Rev. 1-2003 in section 6.4 on 'Post-irradiation verification'.
These include:
- Detection of irradiated food containing fat - Gas chromatographic analysis of hydrocarbons
- Detection of irradiated food containing fat - Gas chromatographic/mass spectrometric analysis of 2-alkylcyclobutanones
- Detection of irradiated food containing bone - Method by ESR spectroscopy
- Detection of irradiated food containing cellulose by ESR spectroscopy
- Thermoluminescence detection of irradiated food from which silicate minerals can be isolated
- Detection of irradiated food containing crystalline sugar by ESR spectroscopy
- Detection of irradiated food using photostimulated luminescence
- Detection of irradiated food using Direct Epifluorescent Filter Technique/Aerobic Plate Count (DEFT/APC) - Screening method
- DNA comet assay for the detection of irradiated foodstuffs - Screening method
- Microbiological screening for irradiated food using LAL/GNB procedures
Food irradiation is slowly gaining consumer acceptance in the US and several other countries, but it is slow to gain support within many parts of Europe, including the UK, where the Food Standards Agency (FSA) recommends no extension of application. Many consumers are initially hostile to irradiation but when the process is explained to them, they become generally more in favour. There is a role for respected professional bodies to inform consumers of the advantages and limitations of the technology.
Many surveys have been carried out (mostly in the USA) to assess consumer attitudes to food irradiation (e.g. Bruhn and Schutz, 1989; Resurreccion et al, 1995; Fox 2002; Nayga et al, 2005). Results have consistently shown that many consumers have misconceptions about the technology and believe that it makes food radioactive. When consumers are given information about the process and a chance to try irradiated products for themselves, they are much more likely to accept the technology. Market trials have also met with success (Pszczola, 1992; Johnson et al 2004; Hamilton et al 2004; Harrison et al 2005).
Consumer acceptance of irradiated foods in the USA is being reinforced by three key-drives; these being (i) growing public awareness of the risks from bacteria in meat (and other food products), (ii) growing levels of educational media coverage on food irradiation and (iii) fear of bioterrorism on centralised food production (Deeley, 2002). A Canadian survey in 2012 indicated that over half of the consumers would buy irradiated food (Eustice and Bruhn, 2012) with the conclusion that “Despite the progress made in the introduction of irradiated foods into the marketplace, many consumers and even highly placed policy-makers around the world are still unaware of the effectiveness, safety, and functional benefits that irradiation can bring to foods. Education and skilled marketing efforts are needed to remedy this lack of awareness.”.
There are well-publicised advantages of irradiating those foods which are known to harbour a wide variety of human pathogens as a means of reducing the incidence of foodborne illness. Despite this, a meeting of the Consumer Committee of the Food Standards Agency in the UK (held on March 2, 2005; Cons Comm DO30/04), agreed that any moves to encourage more irradiation in the UK at this time should not be supported, and this view continues to prevail.
The Food and You Survey conducted by the UK Food Standards Agency in 2012 found that 34% of respondents were aware of food irradiation and that of those aware, 51% reported feeling uneasy about the technology. Awareness differed significantly by age: 19% and 54 % among people aged 16-24 and 55-64 respectively, were aware of the technology. Studies conducted beyond Europe in 2017 indicated that awareness in Australia and New Zealand is low regarding food irradiation and that consumers would generally pay a premium for foods that have not been irradiated. The EU evaluation (EC, 2021) has concluded that considering the low public awareness of the technology and consumer wariness when presented with labelling, it is difficult to characterise the current state of public opinion on this subject.
Food irradiation using ionising radiant energy sources is an important tool in the toolbox to assure food safety, providing it is never applied as a substitute for good manufacturing practices (GMP) in the control of food safety and quality. Safety reviews at the national and global level has confirmed that food irradiation is safe when applied at the prescribed doses and under the permitted conditions defined in published Codex Alimentarius food safety standards and national regulations.
Analytical tests have been developed and validated which are recognised internationally to detect whether foods have been irradiated. These can be applied to enforce requirements for label declarations that food has been irradiated to offer consumer information on the status of the food and support informed choice. Some concerns remain, such as inappropriate use of irradiation to treat unfit foods and uncontrolled applications of incorrect dosages which might impact food safety. There are opportunities for future developments to reduce the use of chemical treatments for pest and pathogen control, and to develop specific applications for machine generated irradiation technologies to provide effective means to ensure food quality and minimise losses and waste without relying on radionuclide sources.
Advisory Committee on Irradiated and Novel Foods (1986). Report on the safety and wholesomeness of irradiated food HMSO: London
Agarwal, S.R. and Sreenivasan, A., 1972. Packaging of irradiated flesh food: A review. J.Food Technol., 8, 27 -37.
Aikawa, Y, 2000, A new facility for X-ray irradiation and its application, Radiat. Phys.Chem. 57, 609612.
Arvanitoyannis I S (Ed.) (2010). Irradiation of Food Commodities: Techniques, Applications, Detection, Legislation, Safety and Consumer Opinion. Academic Press.
Benebion. S.A.FOOD Technology Service Inc. GRAY*STAR, Inc. Food Irradiation Processing Alliance, FIPA is an affiliate of the International Irradiation Association.
Berry Ottaway, P. (Ed.) (2012) The Technology of Vitamins in Food. Springer.
Bhumiratana, N., Belten, L.K. & Bruhn, C.M. (2007) Effect of an educational program on attitudes of California consumers toward food irradiation. Food Protection Trends, 27 (10), 744–748.
Bradley, R. (1984) Radiation Technology Handbook. Marcel and Dekker ,Inc. NY
Bruhn,C.M. and Schutz,H.G. (1989) Consumer Awareness and Outlook for Acceptance of Food Irradiation. Food Technology 43 (7): 93-94,97
Chauhan, S., Kumar, R., Nadanasabapathi, S. and Bawa, A. (2009). Detection Methods for Irradiated Foods—Current Status. Comprehensive Reviews in Food Science and Food Safety. 8. 4 - 16. 2009 10.1111/j.1541-4337.2008.00063.x.
Clegg, D.W. (Ed.) (1991) Irradiation Effects on Polymers. Elsevier Applied Science, New York.
Cleland, M.R., Pageau, G.M., Comparison of X-ray and gamma-ray sources for industrial radiation processes, Nucl. Instrum. Methods, Sect. B 24/25(1987) 967972.
Codex (1991) The Codex Standard for the Labelling of Prepackaged Food (Codex Standard 106-1085 as amended in 1991).
Codex (2003). General standard for irradiated foods. Codex Standard 106-1983, rev.1-2003
DeWit, M. A., Koopmans, M.P., van Duynhoven, Y.T. (2003) Risk factors for norovirus, Sapporo-like virus and Group A rotavirus gastroenteritis. Emerging Inf. Dis. (9)(12), 163-170.
Deeley, C (2002) Food irradiation: setting new standards or a slippery slope? Food Sci. Technol. 16, 52-55.
Diehl, J.F. (1983) Radiolytic effects on foods, in Preservation of Foods by Ionising Radiation, Vol.1, (eds. E.S. Josephson and M.S. Peterson), CRC Press Inc. Boca Raton, Florida, pp.279-357.
Diehl, J.F. (1991) Nutritional effects of combining irradiation with other treatments. Food Control, 2 (1), 20-25.
Diehl, J.F. (1995) Safety of Irradiated Foods 2nd. Ed.Marcel Dekker Inc. NY.
Diehl, J.F. and Josephson, E.S. (1994) Assessment of the wholesomeness of irradiated foods (a review). Acta Alimentaria 23, 195-214.
EFSA (2011). EFSA Statement on the safety of Irradiation of Food, EFSA Journal 2011;9(4): 2107.
EU Scientific Committee on Veterinary Residues Related to Public Health Opinion on “Verotoxigenic E.coli (VTEC) in Foodstuffs: adopted 20-21 January 2003 and posted on the web.
EU (2012). Report from the Commission to the European Parliament and he Council on Food and Food Ingredients Treated with Ionising Radiation or the year 2011, COM(2012) 659 final. http://eur-lex.europa.eu/LexUriServ/LexUriServ.do?uri=COM:2012:0659:FIN:EN:PDF
Eustice R F (2011). Food Irradiation: A Global Perspective and Future Prospects http://www.cirms.org/pdf/2011_conference_pdf/2011_10_18_CIRMS_Eustice.pdf
Eustice R F and Bruhn C M (2012). Consumer Acceptance and Marketing of Irradiated Foods, In Food Irradiation Research and Technology, Second Edition, Eds. C H Sommers and X Fan, IFT Press/Wiley-Blackwell, 173-195).
Ferreira, I.C.F.R.; Antonio, A.L.; Verde S.C. 2017 Food Irradiation Technologies: Concepts, Applications and Outcomes DOI https://doi.org/10.1039/9781788010252
Follet, P.A, Weinert E.D. (2012). Phytosanitary irradiation of fresh tropical commodities in Hawaii: Generic treatments, commercial adoption, and current issues. Rad. Phys. Chem 81, 1064-1067.
Food Standards Agency (UK) 2002. Food Survey for Irradiated Foods – Herbs and Spices, Dietary Supplements and Prawns and Shrimps. Food Survey Information Sheet Number 25/02. June 2002 for Irradiated Foods - Herbs and Spices, Dietary Supplements and Prawns and Shrimps. Food Survey Information Sheet Number 25/02. June 2002.
Food Standards Agency Consumer Committee (2004) Food Irradiation Cons. Comm D030/04.
Food Standards Agency (2010). Good Practice Guide on Compliance with the Legislation on Irradiation of Food Ingredients particularly for Food Supplements .http://www.crnuk.org/uploads/content/irradiation-of-foods-september-2010.pdf
Food Standards Agency (2011). Foodborne Disease Strategy 2010-15 An FSA Programme for the Reduction of Foodborne Disease in the UK. http://www.food.gov.uk/multimedia/pdfs/fds2015.pdf
Fox, J.B., Thayer, D.W., Jenkins, R.K. et al (1989) Effect of gamma irradiation on the B vitamins of pork chops and chicken breasts. Internat. J. Radiat. Biol. 55 689-703
Fox, J.A. (2002) Influences on Purchase of Irradiated Foods Food Technology, 56 (11), 34-37.
Frankhauser, R.L., Monroe, S.S., Noel, J.S.et al. (2002) Epidemiologic and molecular trends of Norwalk-like viruses associated with outbreaks of gastroenteritis in the United States. J. Inf. Dis., July 1, 186 (1) 1-7.
Hale, A., Mattick, K., Lewis, D et al (2000). Distinct epidemiological patterns of Norwalk-like virus infection. J. Med. Virol., Sept. 662(1),99-103.
Hannan, R.S. (1955) Scientific and technological problems in using ionising radiations for the preservation of food. Dept. Sci. Indust. Res. Food Investigation Report No. 61. H.M.S.O.
Hayashi, T., Decontamination of dry food ingredients and seeds with soft-electrons (low-energy electrons), Food Sci. Technol. Int. Tokyo 4 (1998)114120.
IAEA (2010).http://www-naweb.iaea.org/nafa/fep/crp/fep-irradiated-foods-for-ICP-1RCM.pdf
International Consultative Group on Food Irradiation (1991) Facts about Food Irradiation. (set of 14 fact sheets covering all aspects of food irradiation issued as public information). ICGFI Fact Series 1-14. IAEA, Vienna
IFT Scientific Status Summary (2004). Food Technology 58(11).48-55.
Johnson, Adrienne M., Estes Reynolds, A. et al (2004) Consumer Acceptance of Electron-Beam Irradiated Ready-to-Eat Poultry Meats. Food Processing Preservation, 28, 302-319.
Kilcast D (1993). Effects of irradiation on vitamins. Food Chem., 49, 157-64.
Komolprasert, V. (2007). Packaging for Foods Treated by Ionizing Radiation.” In Packaging for Nonthermal Processing of Food, ed. Jung H. Han, pp. 87-116. IFT Press: Blackwell Publishing.
Koopmans, M. and Duizer, E. (2004) Foodborne viruses: an emerging problem. J. Food Microbiol ,(90): 23-4.
McMurray, C.H., Stewart, E.M., Gray, R et al. (1996) Detection methods for irradiated foods – current status. Royal Society of Chemistry Special Publication No.171, Cambridge.
Miller, R.D.(2005) Electronic Irradiation of Foods: An Introduction to the Technology Springer.NY.
Obodovskiy, I. (2019) Chapter 30 - Radiation Sterilization, Editor(s): Ilya Obodovskiy, Radiation, Elsevier, 2019, Pages 373-378, ISBN 9780444639790, https://doi.org/10.1016/B978-0-444-63979-0.00030-6.
Olivieira, C., Salgado, J., Botelho, M.L., Ferreira, L.M., Dose determinations by Monte Carlo calculations, Radiat. Phys. Chem. 57 (2000) 667670.
Osterholm, M.T. and Morgan, A.P.(2004) “The Role of Irradiation in Food Safety New England Journal of Medicine, April 29th.
Pszczola, D.E. (1992) Irradiated Produce reaches Midwest market. Food Technology 46(5), 89-92.
Pszczola, D.E.(1997) 20 ways to market the concept of food irradiation. Food Technology,51, 46-48.
Resurreccion, A.V.A., Galvez, F.C.F., Fletcher, S.M. et al (1995) Consumer attitudes towards irradiated foods: Results of a new study. Journal of Food Protection,58, 193-196.
Roberts, P.B. Food irradiation: Standards, regulations and world-wide trade, Radiation Physics and Chemistry, Volume 129, 2016, Pages 30-34, ISSN 0969-806X, https://doi.org/10.1016/j.radphyschem.2016.06.005.
Satin, M. (1996) Food Irradiation: A Guidebook 2nd.Ed CRC Press, Boca Raton, Fla.
Saylor, M.C., and Jordan, T.M., Application of mathematical modelling technologies to industrial radiation processing. Radiat. Phys. Chem. 57 (2000) 697700.
Schutz, H.G., Bruhn, C and Diaz-Knauf (1989). Consumer Attitude Toward Irradiated Foods: Effects of Labeling and Benefits Information. Food Technology 43(10): 80-86.
Scott Smith, J and Suresh Pillai (2004). Irradiation and Food Safety. Food Technology 58(11) 48-55.
Sommers C H and Fan X (Eds.) (2012). Food Irradiation Research and Technology, Second Edition IFT Press/Wiley-Blackwell.
Stewart, Eileen M.(2004 a) Food irradiation: more pros than cons? Biologist (51) (1) 91-94.
Stewart, Eileen M.(2004 b) Food irradiation: more pros than cons? Part 2. Biologist (51) (2) 141-144.
Suresh, D., Pillai, Leslie A., Braby, Lavergne et al (2005) Electron Beam Technology for Food Irradiation. The International Review of Food Science and Technology (Winter 2004/2005).
Tauxe, Robert V.(2001) Food Safety and Irradiation: Protecting from Foodborne Infections. Emerging Infectious Diseases, 7 (3),June 2001.
Thayer, Donald W.(2004). Irradiation of Food – Helping to Ensure Food Safety. N Engl J Med, 350:1811-1812.
Urbain, W.M. (1986) Food Irradiation. Academic Press Inc. Harcourt Brace Jovanovich Publishers.
Variyar PS, Chatterjee S, Sajilata MG, Singhal RS and Sharma A, (2008). Natural Existence of 2-
Alkylcyclobutanones. Journal of Agricultural and Food Chemistry, 56, 11817-11823.
Wilkinson, V.M. and Gould, G.W. (1996). Food Irradiation: a reference guide. Butterworth Heinemann.
World Health Organisation (1966). The Technical Basis for Legislation on Irradiated Food. WHO Technical Report Series No. 316
World Health Organisation (1981). Report of the Working Party on Irradiation of Food. Joint Expert Committee on the Wholesomeness of Irradiated Food (JEFF). WHO Technical Report Series No. 659
World Health Organisation (1994). Safety and nutritional adequacy of irradiated food WHO,Geneva
World Health Organisation (1999). High-dose irradiation: Wholesomeness of food irradiated with doses above 10 kGy. Report of a FAO/IAEA/WHO Study Group. WHO Technical Report Series No. 890, WHO Geneva.
Ziebkewicz, Hamilton., Lori, S and Penner, Karen P. (2004) Consumers’ Perceptions of Irradiated Ground Beef After Education and Product Exposure. Food Protection Trends, 24(10) 740-745. http://www.crnuk.org/uploads/content/irradiation-of-foods-september-2010.pdf
Special issue on Food Irradiation commemorating József Farkas Edited by Dieter A.E. Ehlermann, Kim M. Morehouse Radiation Physics and Chemistry, Volume 129, 2016, Pages 1-64 (December 2016)
Food Irradiation Technologies: Concepts, Applications and Outcomes Editors: Isabel C F R Ferreira, Amilcar L Antonio, Sandra Cabo Verde 2018 Published by Royal Society Chemistry DOI https://doi.org/10.1039/9781788010252 ISBN 978-1-78262-708-1
International Atomic Energy Agency webpage on Food irradiation https://www.iaea.org/topics/food-irradiation and Manual of Good Practice in Food Irradiation https://www.iaea.org/publications/10801/manual-of-good-practice-in-food-irradiation
ISO 14470:2011 Food irradiation — Requirements for the development, validation and routine control of the process of irradiation using ionizing radiation for the treatment of food (last reviewed 2018) https://www.iso.org/standard/44074.html#:~:text=ISO%2014470%3A2011%20specifies%20requirements,guidelines%20for%20meeting%20the%20requirements.
FAO/WHO Codex Alimentarius GENERAL STANDARD FOR IRRADIATED FOODS 106-1983 Rev.1-2003 https://www.fao.org/fao-who-codexalimentarius/sh-proxy/en/?lnk=1&url=https%253A%252F%252Fworkspace.fao.org%252Fsites%252Fcodex%252FStandards%252FCXS%2B106-1983%252FCXS_106e.pdf
FAO/WHO Codex Alimentarius GENERAL METHODS FOR THE DETECTION OF IRRADIATED FOODS CODEX STAN 231-2001, revised by its 26th Session in 2003 https://www.fao.org/fao-who-codexalimentarius/sh-proxy/en/?lnk=1&url=https%253A%252F%252Fworkspace.fao.org%252Fsites%252Fcodex%252FStandards%252FCXS%2B231-2001%252FCXS_231e.pdf
FAO/WHO Codex Alimentarius Code of Practice for Radiation Processing of Food (CAC/RCP 19-1979) last modified 2033 https://www.fao.org/fao-who-codexalimentarius/sh-proxy/en/?lnk=1&url=https%253A%252F%252Fworkspace.fao.org%252Fsites%252Fcodex%252FStandards%252FCXC%2B19-1979%252FCXP_019e.pdf
Institute of Food Science & Technology has authorised the publication of the following Information Statement on Food Irradiation.
This is an update to the Information Statement that has been prepared in 2013. This Information Statement has been updated, peer-reviewed and approved by the IFST Scientific Committee and is dated November 2022.
The Institute takes every possible care in compiling, preparing and issuing the information contained in IFST Information Statements, but can accept no liability whatsoever in connection with them. Nothing in them should be construed as absolving anyone from complying with legal requirements. They are provided for general information and guidance and to express expert professional interpretation and opinion, on important food-related issues.